Enhanced Roll Porous Scaffold 3D bioprinting technology
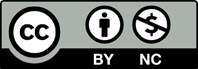
The upgrade of the Roll Porous Scaffold(RPS) 3D bioproduction technique provides significant advantages over today’s dominant analogues. The density 10–15μmcells in the formed object increased up to ~1.26×108cells/mL. The improvement of droplet inkjet methods is achieved by draining excess water through a comb. In addition, a modified foam/lattice-based structure of the scaffold <1%w/vwith a new support ribbon ensures cleaner and more precise biological object shaping. The updated RPS offers not only new hope for overcoming the shortage of organs for transplantation but also rejuvenation of the whole organismdue to the forming of your own endocrine glands. It should be noted that albeit RPS is a project and has never used, it is a derivative of the time-tested methods and components of bioprinting. Potential of RPS for building multicellular tissue of high density and accuracy with a vascular system, with a width of 333mmand a volume >1.7Lper hour at a layer thickness of 18μm.
- Das, S., Gordián-Vélez, W.J., Ledebur, H.C., Mourkioti, F., Rompolas, P., Chen, H.I., Serruya, M.D., & Cullen, D.K. (2020). Innervation: the missing link for biofabricated tissues and organs. NPJ Regen. Med., 5(1), 11. https://doi.org/10.1080/17452759.2017.132513
- Freeman, S., Ramos, R., Chando, P.A., Zhou, L., Reeser, K., Jin, S., Soman, P., & Ye, K. (2019). A bioink blend for rotary 3D bioprinting tissue engineered small-diameter vascular constructs. Acta Biomater., 95, 152–164.
- Jammalamadaka, U., & Tappa, K. (2018). Recent advances in biomaterials for 3D printing and tissue engineering. J. Funct. Biomater., 9(1), 22.
- Karvinen, J., & Kellomäki, M. (2023). Design aspects and characterization of hydrogel-based bioinks for extrusion-based bioprinting. Bioprinting, 32, e00274. https://doi.org/10.1016/j.bprint.2023.e00274
- Kyle, S., Jessop, Z.M., Al-Sabah, A., & Whitaker, I.S. (2017). Printability of candidate biomaterials for extrusion based 3D printing: state-of-the-art. Adv. Healthc. Mater., 6(16), 1700264.
- Leberfinger, A.N., Dinda, S., Wu, Y., Koduru, S.V., Ozbolat, I., Ravnic, D.J., & Ozbolat, I.T. (2019). Bioprinting functional tissues. Acta Biomater., 95, 32–49.
- Li, J., Wu, C., Chu, P.K., & Gelinsky, M. (2020). 3D printing of hydrogels: rational design strategies and emerging biomedical applications. Mater. Sci. Eng. R Rep., 140, 100543.
- Niklason, L.E., & Lawson, J.H. (2020). Bioengineered human blood vessels. Science, 370(6513), eaaw8682.
- Salg, G.A., Blaeser, A., Gerhardus, J.S., Hackert, T., & Kenngott, H.G. (2022). Vascularization in bioartificial parenchymal tissue: bioink and bioprinting strategies. Int. J. Mol. Sci., 23(15), 8589.
- Shulunov, V.R. (2015). The program spiral converting parallel similar objects into a linear sequence [Programma spiral’nogo preobrazovaniya parallel’no podobnykh ob’ektov v linejnuyu posledovatel’nost’]. Certificate of state registration of computer programs No. 2016613199(RU), 21 Dec 2015. (in Russian). doi:10.13140/RG.2.2.17982.05446
- Shulunov, V.R. (2016a). Algorithm for converting 3D objects into rolls using spiral coordinate system. Virtual and Physical Prototyping, 11(2), 91–97. https://doi.org/10.1080/17452759.2016.1175360
- Shulunov, V.R. (2016b). Linear Spiral Convertor for 3D Objects into a Ribbon [Linejno Spiral’nj Convertor slojov3D ob’ektov v lentu]. Certificate of State Registration of Computer Programs No. 2017614132(RU), 07 Dec 2016. (in Russian). doi:10.13140/RG.2.2.29313.25443/1
- Shulunov, V.R. (2016c). The Program Spiral Converting Solids of Revolution into a linear Sequence [Programma Spiral’nogo Preobrazovaniya Tel vraschenia v linejnuyu Posledovatel’nost’]. Certificate of State Registration of Computer Programs No. 2017614186(RU), 26 Oct 2016. (in Russian). doi:10.13140/RG.2.2.15570.35523/1
- Shulunov, V.R. (2017a). Transformation of 3D object into flat ribbon for RPS additive manufacturing technology. Rapid Prototyping Journal, Vol. 23 No. 2, pp. 273-279. doi: 10.1108/RPJ-11-2015-0164.
- Shulunov, V.R. (2017b). Comparison of algorithms for converting 3D objects into rolls, using a spiral coordinate system. Virtual and Physical Prototyping, 12(3), 249–260. https://doi.org/10.1080/17452759.2017.1325132.
- Shulunov, V.R., & Esheeva, I.R. (2017c). Accelerated algorithm for solids of revolution converting into ribbon by spiral coordinate system. International Journal of Intelligent Engineering and Systems, Vol. 10(3), 117–125. doi: 10.22266/ijies2017.0630.13.
- Shulunov, V.R., Esheeva, I.R. (2017d). A linear algorithm for conformal 3D-to-flatness coordinates conversion. Virtual and Physical Prototyping, 12(1), 85–94. https://doi.org/10.1080/17452759.2016.1276820.
- Shulunov, V.R. (2018). Advanced Roll Powder Sintering additive manufacturing technology. Int. J. Interact. Des. Manuf. https://doi.org/10.1007/s12008-018-0475-7.
- Shulunov, V.R. (2019). A novel Roll Porous Scaffold 3D bioprinting technology. Bioprinting, Volume 13, e00042, https://doi.org/10.1016/j.bprint.2019.e00042.
- Shulunov, V.R. (2020). Parallel slicer of STL files for Roll Powder Sintering additive technology [Parallel'nyy slayser STL faylov dlya Roll Powder Sintering additivnoy tekhnologii]. Certificate of State Registration of Computer Programs No. 2020618781 (RU), 15 May 2020. (in Russian). doi:10.13140/RG.2.2.22176.35848.
- Zhang, Y.S., Yue, K., Aleman, J., Mollazadeh-Moghaddam, K., Bakht, S.M., Yang, J., Jia, W., Dell’Erba, V., Assawes, P., Shin, S.R., et al. (2017). 3D bioprinting for tissue and organ fabrication. Ann. Biomed. Eng., 45(1), 148–163.
- Zhang, K., Yan, S., Li, G., Cui, L., & Yin, J. (2015). In-situ birth of MSCs multicellular spheroids in poly (L-glutamic acid)/chitosan scaffold for hyaline-like cartilage regeneration. Biomaterials, 71, 24–34. doi: 10.1016/j.biomaterials.2015.08.037.